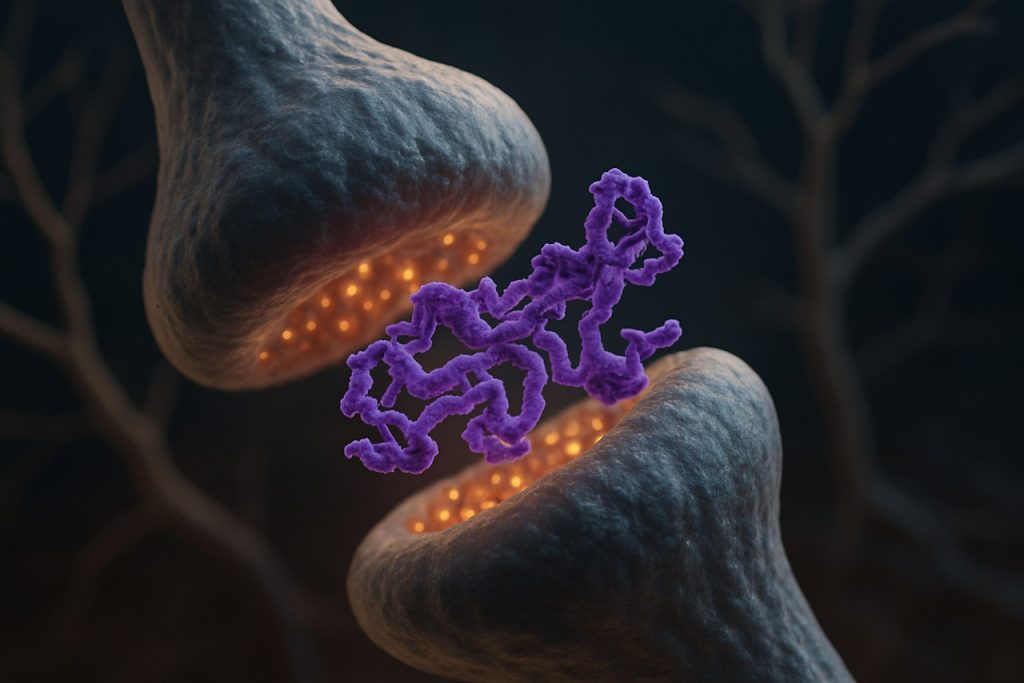
Decoding Neurexin Structure-Function Relationships: How Molecular Architecture Drives Synaptic Precision and Neural Diversity
- Introduction: The Central Role of Neurexins in Synaptic Biology
- Molecular Architecture of Neurexins: Domains and Isoforms
- Alternative Splicing: Generating Neurexin Diversity
- Ligand Interactions: Partners and Binding Mechanisms
- Neurexin-Mediated Synaptic Specification
- Functional Implications in Neural Circuit Assembly
- Neurexin Mutations and Neurological Disorders
- Experimental Approaches to Studying Neurexin Structure-Function
- Emerging Technologies and Structural Insights
- Future Directions: Therapeutic Potential and Unanswered Questions
- Sources & References
Introduction: The Central Role of Neurexins in Synaptic Biology
Neurexins are a family of presynaptic cell adhesion molecules that play a pivotal role in the formation, specification, and maintenance of synapses within the central nervous system. Their structural complexity and functional versatility underpin their centrality in synaptic biology. Neurexins are encoded by three genes—NRXN1, NRXN2, and NRXN3—each of which produces two principal isoforms through alternative promoter usage: the longer α-neurexins and the shorter β-neurexins. This dual-isoform system is further diversified by extensive alternative splicing, resulting in thousands of potential neurexin variants, each with distinct binding affinities and functional properties.
Structurally, α-neurexins are characterized by six laminin/neurexin/sex hormone-binding globulin (LNS) domains interspersed with three epidermal growth factor (EGF)-like repeats, while β-neurexins contain a single LNS domain. Both isoforms share a conserved transmembrane region and a short intracellular tail that interacts with presynaptic scaffolding proteins. The extracellular domains of neurexins mediate highly specific interactions with a variety of postsynaptic partners, most notably neuroligins, but also other molecules such as LRRTMs and cerebellins. These interactions are essential for the alignment of pre- and postsynaptic specializations, thereby orchestrating synaptic transmission and plasticity.
The functional diversity of neurexins is largely attributed to their alternative splicing, which modulates their binding specificity and affinity for different postsynaptic ligands. For example, the presence or absence of specific splice inserts can determine whether a neurexin variant preferentially binds to neuroligins or to other synaptic organizers. This molecular code enables neurexins to contribute to the specification of synaptic properties, such as excitatory versus inhibitory neurotransmission, and to the fine-tuning of synaptic strength and plasticity.
Disruptions in neurexin structure or expression have been implicated in a range of neurodevelopmental and neuropsychiatric disorders, including autism spectrum disorder and schizophrenia. This highlights the critical importance of neurexin structure-function relationships in maintaining synaptic integrity and neural circuit function. Ongoing research, supported by leading neuroscience organizations such as the National Institutes of Health and the National Institute of Neurological Disorders and Stroke, continues to elucidate the molecular mechanisms by which neurexins regulate synaptic biology, offering insights into both normal brain function and disease pathogenesis.
Molecular Architecture of Neurexins: Domains and Isoforms
Neurexins are a family of presynaptic cell adhesion molecules that play a pivotal role in synapse formation and function within the mammalian nervous system. Their molecular architecture is highly modular, enabling diverse interactions with postsynaptic partners and contributing to the specificity and plasticity of synaptic connections. The neurexin gene family comprises three genes—NRXN1, NRXN2, and NRXN3—each of which generates multiple isoforms through alternative promoter usage and extensive alternative splicing. The two principal isoform classes are the longer α-neurexins and the shorter β-neurexins, both of which are type I membrane proteins but differ significantly in their extracellular domain composition.
The extracellular region of α-neurexins is characterized by the presence of six laminin/neurexin/sex hormone-binding globulin (LNS) domains, interspersed with three epidermal growth factor (EGF)-like repeats. This arrangement forms a rod-like structure that provides multiple binding sites for various synaptic ligands, such as neuroligins, cerebellins, and dystroglycan. In contrast, β-neurexins possess a much shorter extracellular segment, containing only the sixth LNS domain and lacking the EGF-like repeats, which restricts their binding repertoire but allows for distinct functional roles at the synapse.
Alternative splicing at several canonical sites within neurexin transcripts further diversifies the extracellular domain structure, modulating ligand-binding affinities and specificities. For example, the presence or absence of inserts at splice site 4 (SS4) in the LNS6 domain critically influences neurexin’s ability to interact with neuroligins and other postsynaptic partners. This splicing-dependent molecular diversity is thought to underlie the fine-tuning of synaptic properties and the establishment of neural circuit specificity.
The intracellular domain of neurexins, though relatively short, contains motifs that interact with presynaptic scaffolding proteins and signaling molecules, thereby linking extracellular adhesion events to intracellular signaling cascades and synaptic vesicle release machinery. The transmembrane region anchors neurexins to the presynaptic membrane, ensuring their strategic localization at active zones.
The structural complexity and isoform diversity of neurexins are essential for their function as synaptic organizers. Disruptions in neurexin structure or expression have been implicated in a range of neurodevelopmental and neuropsychiatric disorders, highlighting the importance of their precise molecular architecture for normal brain function. Research into neurexin structure-function relationships continues to be a major focus for organizations such as the National Institutes of Health and the Nature Publishing Group, which support and disseminate advances in neuroscience and molecular biology.
Alternative Splicing: Generating Neurexin Diversity
Neurexins are a family of presynaptic cell adhesion molecules that play a pivotal role in synapse formation and function within the mammalian nervous system. A key feature underlying the remarkable functional diversity of neurexins is their extensive alternative splicing. The three neurexin genes (NRXN1, NRXN2, and NRXN3) each produce two principal isoforms—α-neurexins and β-neurexins—through the use of alternative promoters. Beyond this, alternative splicing at up to six canonical sites (termed SS1–SS6) within each gene further expands the repertoire of neurexin variants, resulting in thousands of potential isoforms with distinct extracellular domains.
Alternative splicing modulates the inclusion or exclusion of specific exons, thereby altering the protein domains present in each neurexin molecule. This process is highly regulated both spatially and temporally, allowing neurons to fine-tune neurexin expression profiles according to developmental stage, cell type, and synaptic context. The resulting structural diversity is critical for the ability of neurexins to interact with a wide array of postsynaptic ligands, such as neuroligins, LRRTMs, and cerebellins, each of which may preferentially bind to specific neurexin splice variants. For example, the presence or absence of an insert at splice site 4 (SS4) can dramatically influence binding affinity for neuroligins, thereby affecting synaptic specificity and function.
The functional consequences of neurexin alternative splicing are profound. By generating a molecular code at the synaptic cleft, alternative splicing enables neurexins to specify synaptic properties, such as excitatory versus inhibitory identity, synaptic strength, and plasticity. Disruption of neurexin splicing patterns has been implicated in a range of neurodevelopmental and neuropsychiatric disorders, including autism spectrum disorder and schizophrenia, underscoring the importance of precise splicing regulation for normal brain function.
Research into neurexin alternative splicing has been advanced by organizations such as the National Institutes of Health and the Nature Publishing Group, which have supported and disseminated key studies elucidating the molecular mechanisms and functional outcomes of neurexin diversity. The ongoing exploration of neurexin splicing not only enhances our understanding of synaptic biology but also offers potential avenues for therapeutic intervention in synaptic disorders.
Ligand Interactions: Partners and Binding Mechanisms
Neurexins are a family of presynaptic cell adhesion molecules that play a pivotal role in synapse formation and function within the central nervous system. Their ability to interact with a diverse array of ligands underpins their functional versatility and is central to their role in synaptic specification and plasticity. The ligand interactions of neurexins are dictated by their complex structural organization, which includes extensive alternative splicing and the presence of multiple extracellular domains.
Neurexins are encoded by three genes (NRXN1, NRXN2, and NRXN3), each producing longer α-neurexins and shorter β-neurexins through alternative promoter usage. The extracellular region of α-neurexins contains six laminin/neurexin/sex hormone-binding globulin (LNS) domains interspersed with three epidermal growth factor (EGF)-like repeats, while β-neurexins possess a single LNS domain. These LNS domains are the primary sites for ligand binding, and their structural diversity—further expanded by alternative splicing at several canonical sites—enables neurexins to engage in highly specific interactions with a variety of postsynaptic partners.
One of the most well-characterized neurexin ligands is neuroligin, a postsynaptic cell adhesion molecule. The neurexin-neuroligin interaction is calcium-dependent and is mediated by the LNS6 domain of neurexins. This binding is highly regulated by alternative splicing at splice site 4 (SS4) in neurexins, which can either permit or prevent neuroligin binding, thereby modulating synaptic specificity and function. Other prominent neurexin ligands include leucine-rich repeat transmembrane proteins (LRRTMs), cerebellins, and dystroglycan. Each of these ligands recognizes distinct neurexin isoforms or splice variants, contributing to the molecular diversity of synaptic connections.
The binding mechanisms between neurexins and their partners often involve precise molecular recognition, with structural studies revealing that the LNS domains form specific interfaces with ligand proteins. For example, the interaction with LRRTMs is also regulated by alternative splicing, and the presence or absence of specific inserts can dramatically alter binding affinity. Additionally, cerebellins act as synaptic organizers by bridging neurexins and postsynaptic glutamate receptor delta subunits, further illustrating the modular and combinatorial nature of neurexin-mediated adhesion.
The functional consequences of these ligand interactions are profound, influencing synaptic assembly, neurotransmitter receptor localization, and synaptic plasticity. Disruptions in neurexin-ligand binding have been implicated in neurodevelopmental disorders, including autism spectrum disorders and schizophrenia, highlighting the clinical significance of understanding these molecular mechanisms. Ongoing research by organizations such as the National Institutes of Health and the Nature Publishing Group continues to elucidate the structural basis and physiological relevance of neurexin-ligand interactions, advancing our knowledge of synaptic biology.
Neurexin-Mediated Synaptic Specification
Neurexins are a family of presynaptic cell adhesion molecules that play a pivotal role in synaptic specification, the process by which synapses acquire distinct functional properties. The structure-function relationships of neurexins are central to their ability to mediate diverse synaptic connections in the mammalian brain. Neurexins are encoded by three genes (NRXN1, NRXN2, and NRXN3), each producing two principal isoforms: the longer α-neurexins and the shorter β-neurexins, generated by alternative promoters and extensive alternative splicing. This molecular diversity underpins the functional versatility of neurexins at synapses.
Structurally, α-neurexins contain six laminin/neurexin/sex hormone-binding globulin (LNS) domains interspersed with three epidermal growth factor (EGF)-like repeats, while β-neurexins possess a single LNS domain. Both isoforms share a conserved transmembrane region and a short cytoplasmic tail that interacts with intracellular scaffolding proteins. The extracellular domains of neurexins are responsible for binding to a variety of postsynaptic ligands, including neuroligins, leucine-rich repeat transmembrane proteins (LRRTMs), and cerebellins, which are critical for synapse formation and specification.
Alternative splicing at multiple sites, particularly at splice site 4 (SS4), generates thousands of neurexin variants, each with distinct ligand-binding properties. This splicing-dependent diversification allows neurexins to selectively interact with specific postsynaptic partners, thereby influencing the molecular identity and functional properties of individual synapses. For example, the presence or absence of an insert at SS4 can determine whether a neurexin variant binds to neuroligins or LRRTMs, thus specifying excitatory or inhibitory synaptic connections.
Functionally, neurexins act as synaptic organizers by bridging the presynaptic and postsynaptic membranes and recruiting synaptic machinery. Their interactions with postsynaptic ligands trigger the assembly of synaptic specializations, regulate neurotransmitter receptor localization, and modulate synaptic transmission. Disruption of neurexin structure or expression has been implicated in neurodevelopmental disorders such as autism spectrum disorder and schizophrenia, highlighting the importance of precise neurexin-mediated signaling for normal brain function.
Research into neurexin structure-function relationships continues to elucidate how molecular diversity at the neurexin locus translates into the vast heterogeneity of synaptic types and functions observed in the brain. This knowledge is foundational for understanding the molecular logic of synaptic specification and the pathogenesis of synaptic disorders. For further information, authoritative resources such as the National Institutes of Health and the Nature Publishing Group provide comprehensive overviews and primary research articles on neurexin biology.
Functional Implications in Neural Circuit Assembly
Neurexins are a family of presynaptic cell adhesion molecules that play a pivotal role in the assembly and specification of neural circuits. Their structure-function relationships are central to understanding how synaptic connections are formed, maintained, and diversified in the mammalian brain. Neurexins are encoded by three genes (NRXN1, NRXN2, and NRXN3), each producing multiple isoforms through alternative promoter usage and extensive alternative splicing. The two principal isoforms, α-neurexins and β-neurexins, differ in their extracellular domain composition, which directly influences their binding affinities and functional roles at synapses.
Structurally, α-neurexins are characterized by six laminin-neurexin-sex hormone-binding globulin (LNS) domains interspersed with three epidermal growth factor (EGF)-like repeats, while β-neurexins contain only a single LNS domain. This modular architecture enables neurexins to interact with a diverse array of postsynaptic partners, including neuroligins, leucine-rich repeat transmembrane proteins (LRRTMs), and cerebellins. The specificity and strength of these interactions are further modulated by alternative splicing at several canonical sites, most notably at splice site 4 (SS4), which can dramatically alter ligand binding profiles and downstream signaling.
Functionally, neurexins act as molecular codes that instruct synapse formation and identity. Their extracellular domains mediate trans-synaptic adhesion, ensuring precise alignment of pre- and postsynaptic specializations. This adhesion is not merely structural; it triggers intracellular signaling cascades that regulate synaptic differentiation, neurotransmitter receptor recruitment, and synaptic plasticity. For example, the presence or absence of an insert at SS4 can determine whether a neurexin isoform preferentially binds neuroligins or LRRTMs, thereby influencing whether a synapse becomes excitatory or inhibitory.
The functional diversity generated by neurexin alternative splicing is essential for the fine-tuning of neural circuits. Disruption of neurexin structure or splicing patterns has been implicated in a range of neurodevelopmental and neuropsychiatric disorders, including autism spectrum disorder and schizophrenia. This highlights the critical role of neurexin structure-function relationships in both normal brain development and disease pathogenesis. Ongoing research, supported by organizations such as the National Institutes of Health and the National Institute of Neurological Disorders and Stroke, continues to elucidate the molecular mechanisms by which neurexins orchestrate neural circuit assembly and function.
Neurexin Mutations and Neurological Disorders
Neurexins are a family of presynaptic cell adhesion molecules that play a pivotal role in the formation, specification, and maintenance of synapses in the mammalian nervous system. Structurally, neurexins are encoded by three genes—NRXN1, NRXN2, and NRXN3—each of which produces two principal isoforms through alternative promoter usage: the longer α-neurexins and the shorter β-neurexins. These isoforms differ in their extracellular domains, with α-neurexins containing six laminin/neurexin/sex hormone-binding globulin (LNS) domains interspersed with three epidermal growth factor (EGF)-like repeats, while β-neurexins possess a single LNS domain. Both isoforms share a common transmembrane region and a short intracellular tail that interacts with presynaptic scaffolding proteins.
The structural diversity of neurexins is further amplified by extensive alternative splicing at multiple canonical sites, generating thousands of distinct neurexin variants. This molecular heterogeneity enables neurexins to engage in highly specific interactions with a variety of postsynaptic partners, such as neuroligins, leucine-rich repeat transmembrane proteins (LRRTMs), and cerebellins. These interactions are essential for synaptic adhesion, alignment of pre- and postsynaptic specializations, and the regulation of synaptic transmission and plasticity.
Functionally, neurexins act as molecular organizers of synapses, influencing both excitatory and inhibitory synaptic connectivity. The specific combination of neurexin isoforms and splice variants expressed at a given synapse determines the repertoire of postsynaptic ligands that can be recruited, thereby modulating synaptic properties such as neurotransmitter release probability, receptor composition, and synaptic strength. For example, alternative splicing at site 4 of NRXN1 can regulate the binding affinity for neuroligins, which in turn affects the balance between excitatory and inhibitory synapses.
Disruption of neurexin structure—whether through genetic mutations, deletions, or aberrant splicing—can impair synaptic function and has been implicated in a range of neurodevelopmental and neuropsychiatric disorders, including autism spectrum disorder, schizophrenia, and intellectual disability. The critical role of neurexins in synaptic organization and function is underscored by studies demonstrating that even subtle alterations in neurexin structure can lead to profound changes in neural circuitry and behavior. Ongoing research by leading neuroscience organizations, such as the National Institutes of Health and the National Institute of Neurological Disorders and Stroke, continues to elucidate the precise structure-function relationships of neurexins and their relevance to human brain health.
Experimental Approaches to Studying Neurexin Structure-Function
Understanding the structure-function relationships of neurexins is fundamental to elucidating their roles in synaptic organization and neuropsychiatric disorders. Neurexins are a family of presynaptic cell adhesion molecules characterized by extensive alternative splicing, resulting in thousands of isoforms with distinct binding properties and functions. Experimental approaches to studying neurexin structure-function relationships integrate molecular biology, structural biology, biochemistry, and advanced imaging techniques.
One foundational approach involves the use of X-ray crystallography and cryo-electron microscopy (cryo-EM) to resolve the three-dimensional structures of neurexin extracellular domains and their complexes with postsynaptic ligands, such as neuroligins and LRRTMs. These high-resolution structures have revealed the modular architecture of neurexins, including their LNS (laminin, neurexin, sex hormone-binding globulin) domains, EGF-like repeats, and the impact of alternative splicing on ligand binding. For example, the presence or absence of specific splice inserts at defined sites can dramatically alter the affinity and specificity of neurexin-ligand interactions, as demonstrated in structural studies by leading neuroscience research institutes and consortia.
Site-directed mutagenesis is another critical experimental strategy. By introducing targeted mutations into neurexin genes or proteins, researchers can dissect the functional contributions of individual domains or amino acid residues. These mutant constructs are often expressed in heterologous systems or neurons, followed by biochemical assays—such as co-immunoprecipitation or surface plasmon resonance—to quantify binding affinities and map interaction interfaces. Such studies have clarified how specific structural features of neurexins govern their ability to organize synaptic specializations and recruit synaptic proteins.
Genetic manipulation in model organisms, including mice and zebrafish, provides in vivo context for neurexin structure-function analysis. Conditional knockout or knock-in models allow researchers to assess the physiological consequences of deleting or modifying neurexin isoforms or domains. Electrophysiological recordings and behavioral assays in these models have linked neurexin structural diversity to synaptic transmission, plasticity, and animal behavior, offering insights into the molecular basis of neurodevelopmental and neuropsychiatric disorders.
Advanced imaging techniques, such as super-resolution microscopy and single-molecule tracking, further enable visualization of neurexin distribution, clustering, and dynamics at synapses. Combined with fluorescently tagged neurexin constructs, these approaches reveal how structural variations influence neurexin localization and interactions in living neurons.
Collectively, these experimental approaches—spanning structural, biochemical, genetic, and imaging methodologies—have been instrumental in unraveling the complex structure-function relationships of neurexins. Ongoing research, supported by organizations such as the National Institutes of Health and the Howard Hughes Medical Institute, continues to advance our understanding of how neurexin structural diversity underpins synaptic specificity and brain function.
Emerging Technologies and Structural Insights
Neurexins are a family of presynaptic cell adhesion molecules that play a pivotal role in synapse formation, specification, and function within the central nervous system. The structural complexity of neurexins underpins their diverse functional repertoire, and recent technological advances have significantly deepened our understanding of these relationships. Neurexins are encoded by three genes (NRXN1, NRXN2, NRXN3), each producing multiple isoforms through alternative promoter usage and extensive alternative splicing. The two principal isoforms, α-neurexins and β-neurexins, differ in their extracellular domain architecture, with α-neurexins containing six laminin/neurexin/sex hormone-binding globulin (LNS) domains interspersed with three epidermal growth factor (EGF)-like repeats, while β-neurexins possess a shorter extracellular region with a single LNS domain. This modular structure enables neurexins to engage in a wide array of protein-protein interactions, particularly with postsynaptic partners such as neuroligins, LRRTMs, and cerebellins, thereby orchestrating synaptic specificity and signaling.
Emerging technologies, particularly high-resolution cryo-electron microscopy (cryo-EM) and X-ray crystallography, have provided unprecedented insights into the three-dimensional organization of neurexin domains and their complexes. These structural studies have revealed how alternative splicing at specific sites (notably at splice site 4, or SS4) modulates the binding affinity and selectivity of neurexins for their ligands, effectively acting as a molecular switch that tunes synaptic properties. For example, the presence or absence of an insert at SS4 can determine whether a neurexin isoform preferentially binds neuroligins or other synaptic organizers, influencing excitatory versus inhibitory synapse formation. Such findings underscore the importance of structural diversity in neurexin-mediated synaptic specification.
In addition to static structural snapshots, single-molecule imaging and super-resolution microscopy have enabled visualization of neurexin distribution and dynamics at the synaptic membrane, revealing their nanoscale organization and mobility. These approaches, combined with proteomic mapping and genome editing technologies such as CRISPR/Cas9, have facilitated the dissection of neurexin interactomes and the functional consequences of disease-associated mutations. Notably, mutations and deletions in neurexin genes have been implicated in neurodevelopmental disorders, including autism spectrum disorder and schizophrenia, highlighting the clinical relevance of understanding neurexin structure-function relationships.
The integration of structural biology, advanced imaging, and genetic engineering continues to illuminate the molecular logic by which neurexins orchestrate synaptic connectivity and plasticity. Ongoing efforts by leading research institutions and international consortia, such as the National Institutes of Health and the European Molecular Biology Laboratory, are accelerating the translation of these insights into therapeutic strategies for synaptic dysfunction.
Future Directions: Therapeutic Potential and Unanswered Questions
The intricate structure-function relationships of neurexins have profound implications for future therapeutic strategies and highlight several unanswered questions in neuroscience. Neurexins are presynaptic cell adhesion molecules that play a pivotal role in synapse formation, specification, and maintenance. Their extensive alternative splicing and post-translational modifications generate thousands of isoforms, enabling highly specific interactions with diverse postsynaptic partners such as neuroligins, LRRTMs, and cerebellins. This molecular diversity underpins the functional heterogeneity of synapses across different brain regions and developmental stages.
A major future direction involves leveraging the structural complexity of neurexins to develop targeted therapies for neuropsychiatric and neurodevelopmental disorders. Mutations and copy number variations in neurexin genes (NRXN1, NRXN2, NRXN3) have been strongly associated with conditions such as autism spectrum disorder, schizophrenia, and intellectual disability. Understanding how specific neurexin isoforms contribute to synaptic function and dysfunction could inform the design of precision medicines that restore or modulate neurexin-mediated signaling. For example, small molecules or biologics that enhance or inhibit particular neurexin-ligand interactions may offer novel approaches to correct synaptic deficits in these disorders.
Despite significant advances, several unanswered questions remain. The precise mechanisms by which neurexin structural variants dictate synaptic specificity and plasticity are not fully understood. It is unclear how the combinatorial expression of neurexin isoforms and their dynamic regulation during development and in response to activity contribute to circuit assembly and remodeling. Additionally, the interplay between neurexins and other synaptic organizers, as well as their roles outside the central nervous system, require further elucidation.
Emerging technologies such as single-cell transcriptomics, super-resolution imaging, and genome editing are expected to accelerate discoveries in this field. These tools will enable researchers to map neurexin isoform expression at unprecedented resolution, visualize their nanoscale organization at synapses, and manipulate their function in vivo. Collaborative efforts by leading research institutions and organizations such as the National Institutes of Health and the National Institute of Neurological Disorders and Stroke are driving large-scale studies to unravel the complexities of neurexin biology and its relevance to human disease.
In summary, the future of neurexin research lies in translating structural insights into therapeutic interventions, while addressing fundamental questions about their diverse roles in synaptic organization and brain function. Continued interdisciplinary research will be essential to unlock the full therapeutic potential of targeting neurexin structure-function relationships.
Sources & References
- National Institutes of Health
- Nature Publishing Group
- Howard Hughes Medical Institute
- European Molecular Biology Laboratory