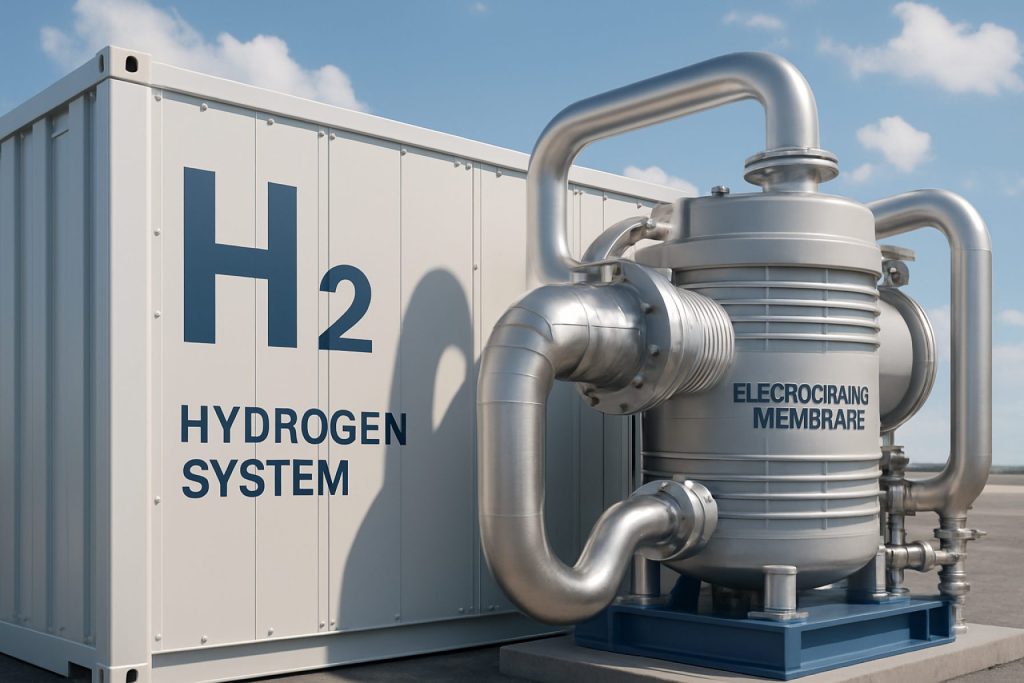
Table of Contents
- Executive Summary: 2025 Outlook and Strategic Implications
- Technology Overview: How Electroceramic Membrane Hydrogen Separation Works
- Key Manufacturers, Suppliers, and Innovators (with Official Sources)
- Market Size and Growth Forecast: 2025–2030
- Competitive Landscape: Major Players and Partnerships
- Application Segments: Energy, Industry, and Mobility
- Performance Metrics: Efficiency, Scalability, and Cost
- Challenges and Barriers to Widespread Adoption
- Regulatory Environment and Industry Standards
- Future Trends: Innovations, Investments, and Long-term Outlook
- Sources & References
Executive Summary: 2025 Outlook and Strategic Implications
Electroceramic membrane hydrogen separation systems are emerging as a pivotal technology in the global transition to low-carbon hydrogen production and utilization. As of 2025, these systems—using mixed ionic-electronic conducting (MIEC) ceramics—are gaining traction for their ability to selectively separate hydrogen from gas mixtures at elevated temperatures, offering potential improvements in efficiency, purity, and operational cost over traditional pressure swing adsorption (PSA) or cryogenic processes.
Several industry leaders have advanced pilot and demonstration projects, signaling growing commercial interest. Topsoe has developed its proprietary SOEC (Solid Oxide Electrolysis Cell) and ceramic membrane technologies for hydrogen and syngas separation, with pilot installations validating high hydrogen purity (>99.9%) and robust operation at industrial scale. Ceramatec, a subsidiary of CoorsTek, continues to scale up its tubular ceramic membrane modules, targeting both hydrogen production and purification from challenging feedstocks, including biomass and industrial off-gases.
On the materials front, advances in perovskite and other oxide-based membrane compositions are improving both hydrogen flux and chemical stability, addressing key durability concerns. Sumitomo Chemical and NGK Insulators are actively developing ceramic membranes for deployment in chemical plants and refineries, with demonstration projects aimed at integrating hydrogen separation into ammonia and methanol production processes.
The strategic implications for 2025 and beyond are significant. Electroceramic membrane systems offer the ability to co-produce hydrogen and high-value chemicals from fossil or renewable sources with integrated CO2 capture, supporting decarbonization goals. They are also being evaluated in conjunction with existing blue hydrogen and carbon capture facilities, as seen in collaborations between Shell and technology partners to test next-generation membrane reactors in industrial settings.
- By 2025, commercial pilots are expected to expand across Asia, Europe, and North America, driven by government incentives for low-carbon hydrogen and stricter emissions targets.
- Ongoing scale-up efforts are focused on reducing capital costs and improving membrane lifespans to compete with incumbent separation technologies.
- Integration with renewable electricity and fluctuating power sources is an active area of R&D, as electroceramic membranes are suited for dynamic operation in Power-to-X applications.
The outlook for electroceramic membrane hydrogen separation systems in the next several years is one of cautious optimism, with the technology advancing towards wider industrial demonstration. Strategic partnerships between membrane developers, chemical producers, and energy majors are expected to accelerate commercialization, positioning electroceramic membranes as a key enabler of sustainable hydrogen value chains.
Technology Overview: How Electroceramic Membrane Hydrogen Separation Works
Electroceramic membrane hydrogen separation systems leverage advanced ceramic materials to selectively extract hydrogen from mixed gas streams, offering a promising alternative to conventional pressure swing adsorption (PSA) or cryogenic techniques. The core mechanism relies on dense, non-porous ceramic membranes—often based on perovskite or mixed proton-electron conducting oxides—that facilitate hydrogen transport via a solid-state mechanism at elevated temperatures (typically 400–900°C). When a hydrogen-containing gas mixture contacts one side of the membrane, hydrogen molecules dissociate into protons and electrons. These protons traverse the ceramic lattice, driven by a chemical potential gradient, and recombine with electrons on the permeate side to form high-purity hydrogen gas.
Recent advancements have centered on improving membrane stability, hydrogen flux, and scalability. Companies such as Haldor Topsoe and Fraunhofer Society are actively developing robust ceramic compositions, including barium cerate and zirconate perovskites, as well as optimizing reactor designs for industrial-scale hydrogen production. For instance, Haldor Topsoe has reported progress on ceramic membrane reactors capable of integrating hydrogen separation directly with processes like steam methane reforming, thereby enhancing overall process efficiency and reducing carbon emissions.
Operational data from recent pilot demonstrations suggests that electroceramic membrane systems can achieve hydrogen purities exceeding 99.9% with flux rates in the range of 0.1–1.0 Nm³/m²h at 600–800°C, depending on membrane composition and system integration. These systems are particularly attractive for decentralised hydrogen production, integration with ammonia or methanol synthesis, and biomass conversion, where process intensification and smaller footprints are valued.
Looking ahead to 2025 and the next few years, several industry players are targeting scale-up and commercialization milestones. The Fraunhofer Society is coordinating European consortia to bring demonstration units to industrial partners, aiming for multi-kW to MW-scale operations. Similarly, Haldor Topsoe expects to validate membrane module performance in real-world chemical plant environments by 2025. Long-term outlooks focus on further membrane durability improvements, cost reduction through manufacturing scale, and system integration with renewable energy sources for low-carbon hydrogen supply.
As industry and policy momentum gathers around clean hydrogen, electroceramic membrane separation stands out as a key enabling technology, with commercialization and performance breakthroughs anticipated within the next few years.
Key Manufacturers, Suppliers, and Innovators (with Official Sources)
As the global demand for clean hydrogen intensifies, electroceramic membrane hydrogen separation systems are attracting significant investment and industrial focus. These systems, often based on perovskite-type or mixed ionic–electronic conducting ceramics, promise high-selectivity hydrogen separation at elevated temperatures—enabling integration with industrial processes and renewable energy sources. The current (2025) landscape highlights several leading manufacturers, suppliers, and innovators pushing the field from lab-scale breakthroughs to commercial deployment.
- Elcogen: Based in Estonia and Finland, Elcogen is a recognized supplier of advanced ceramic cells and stacks, primarily for solid oxide fuel cells, but their expertise in solid oxide technology and materials positions them as a key participant in the transition to membrane-based hydrogen production and separation. Their partnerships with large-scale hydrogen projects indicate growing involvement in the sector.
- CerPoTech: Norwegian company CerPoTech manufactures high-purity ceramic powders such as perovskites, central to the development of electroceramic membranes. Their materials are widely used in R&D and pilot projects for hydrogen separation membranes.
- Saint-Gobain: Through its Ceramics division, Saint-Gobain develops and supplies advanced ceramic materials for a range of applications, including gas separation membranes. Their focus on scalable ceramic manufacturing processes aligns with the anticipated ramp-up of electroceramic membrane deployment in hydrogen purification.
- Fraunhofer IKTS: As part of the Fraunhofer Institute for Ceramic Technologies and Systems, Fraunhofer IKTS leads several demonstration projects utilizing ceramic membranes for hydrogen production and separation, including partnerships with industry for upscaling and integration into chemical plants.
- CoorsTek: American ceramics specialist CoorsTek supplies advanced ceramic components for energy applications, including membranes for high-temperature gas separation. They are expanding their portfolio to support emerging hydrogen markets, with a particular emphasis on durability and manufacturability.
- SOLIDpower: Italian-German company SOLIDpower is recognized for its solid oxide technology and is actively researching membrane-based systems for hydrogen separation and fuel cell hybridization.
The outlook for 2025 and beyond features strong collaboration between material suppliers, membrane developers, and end-users, especially in Europe and Asia. Several pilot plants and demonstration projects are scheduled for operation, targeting both pure hydrogen production and integration with ammonia, methanol, and steel manufacturing sectors. With regulatory drivers accelerating low-emission hydrogen adoption, these organizations are poised to play pivotal roles in scaling up electroceramic membrane technology for industrial hydrogen supply chains.
Market Size and Growth Forecast: 2025–2030
The global market for electroceramic membrane hydrogen separation systems is poised for significant expansion between 2025 and 2030, driven by increasing demand for low-carbon hydrogen production and growing governmental commitments to clean energy transitions. Electroceramic membranes, particularly those based on perovskite and mixed ionic-electronic conducting (MIEC) materials, are attracting attention for their high hydrogen selectivity, thermal stability, and potential integration in industrial-scale processes.
As of 2025, several industry leaders and innovators are scaling up pilot and demonstration plants to validate the commercial viability of these systems. For example, Topsoe is actively developing ceramic membrane technologies for hydrogen production and separation, targeting applications in ammonia plants, refineries, and green hydrogen hubs. Similarly, Haldor Topsoe continues to invest in solid oxide electrolyzer cells (SOECs) and related ceramic membrane advancements to improve hydrogen purity and system efficiencies.
In Europe, the deployment of electroceramic membrane systems is closely aligned with the European Union’s hydrogen strategy. Organizations such as Clean Hydrogen Partnership are supporting demonstration projects and establishing funding mechanisms to accelerate commercial adoption through the late 2020s. This coordinated approach is resulting in increased installations at industrial clusters and chemical plants, with several multi-megawatt-scale pilots slated for commissioning by 2027.
On the technology supplier side, companies like Ceramatec and Oxyn are collaborating with energy majors and industrial gas producers to bring advanced ceramic membrane modules to market. These modules promise hydrogen recovery rates of up to 99% and operational lifetimes exceeding 20,000 hours under industrial conditions.
Market analysts anticipate a compound annual growth rate (CAGR) exceeding 20% for electroceramic membrane hydrogen separation systems during 2025–2030, with market value projections reaching several hundred million USD by the decade’s end. Key growth drivers include expanding green and blue hydrogen projects, stricter emissions regulations, and the need for efficient, scalable hydrogen purification technologies.
- Strategic partnerships and joint ventures between membrane developers and industrial end-users are expected to accelerate market penetration.
- Asia-Pacific, led by Japan and South Korea, is emerging as a high-growth region due to national hydrogen roadmaps and investments in next-generation hydrogen infrastructure.
- R&D efforts are focused on reducing system costs, improving membrane durability, and scaling up production capabilities to meet anticipated demand surges.
Overall, the outlook for electroceramic membrane hydrogen separation systems from 2025 to 2030 is robust, with escalating deployment in both established and emerging hydrogen markets worldwide.
Competitive Landscape: Major Players and Partnerships
The competitive landscape for electroceramic membrane hydrogen separation systems in 2025 is marked by active participation from established industrial players, emerging technology companies, and strategic partnerships aimed at scaling up and commercializing novel membrane technologies. The sector is primarily driven by the increasing demand for high-purity hydrogen, the need for efficient carbon capture and utilization, and global decarbonization initiatives targeting hard-to-abate sectors.
Among major players, Topsoe stands out with its development of solid oxide electrolysis and ceramic membrane reactors. Topsoe’s investment in electroceramic-based hydrogen production is reinforced by collaborations with industry partners to deliver modular, scalable solutions for green hydrogen and ammonia projects. In 2024, Topsoe announced new demonstration projects in Europe, targeting commercial deployment in the 2025–2027 window.
Meanwhile, Kyocera Corporation leverages its advanced ceramics expertise to produce dense and porous ceramic membranes. Kyocera’s recent focus has been on the upscaling of proton-conducting ceramic membranes for both hydrogen separation and fuel cell applications, with ongoing pilot partnerships in Japan and the EU.
Another influential entity is Air Liquide, which has accelerated its R&D and investment in membrane-based hydrogen purification and recovery systems. Air Liquide is collaborating with technology developers to integrate ceramic membrane modules into its global hydrogen infrastructure, aiming for operational pilot systems by late 2025.
On the technology development front, Ceramatec, Inc. continues to advance high-temperature ceramic membrane separators. The company has received funding from government and industrial partners to demonstrate its proton-conducting electroceramic membranes at pilot scale, with a focus on process intensification for refineries and chemical plants.
Strategic partnerships are shaping the competitive environment. In 2024, Siemens Energy and Topsoe announced a collaboration to integrate ceramic membrane technology into large-scale hydrogen production plants. Additionally, Shell is exploring joint ventures with membrane developers to embed high-selectivity electroceramic modules in their blue and green hydrogen projects.
Looking ahead, the next several years will likely see intensifying collaboration between materials specialists, engineering firms, and major hydrogen consumers. The sector is expected to progress from pilot and demonstration projects to early commercial deployments, especially in regions with strong policy support and hydrogen infrastructure investment.
Application Segments: Energy, Industry, and Mobility
Electroceramic membrane hydrogen separation systems are poised to play a transformative role across key application segments—energy, industry, and mobility—in 2025 and the immediate years ahead. These systems leverage mixed ionic-electronic conducting (MIEC) materials and perovskite-type ceramics to selectively separate hydrogen at high temperatures, often above 500°C, offering significant efficiency and purity benefits over conventional technologies.
Energy Sector: The decarbonization of power generation and energy storage has accelerated the deployment of hydrogen infrastructure, with electroceramic membranes increasingly integrated into high-temperature processes such as solid oxide electrolysis and hydrogen-fueled turbines. Companies like Siemens Energy and Bosch are advancing solid oxide cell (SOC) platforms that include hydrogen separation capabilities, aiming for commercial-scale deployment by 2025-2026. These membranes enable more efficient hydrogen extraction from syngas and biomass-derived streams, contributing to the flexible operation of power plants and grid balancing through green hydrogen production.
Industrial Applications: In hard-to-abate sectors such as steel, ammonia, and chemicals, electroceramic membranes are being piloted to recover hydrogen from off-gases or integrate into process loops. Topsoe has announced demonstration-scale projects using their proprietary ceramic membrane technology for hydrogen separation and purification in ammonia plants, targeting improved energy efficiency and emissions reductions. The high selectivity and thermal stability of electroceramic membranes allow for direct integration into industrial reactors, reducing the need for multi-step purification and compression.
Mobility and Transportation: The rollout of fuel cell vehicles and hydrogen refueling infrastructure is driving demand for compact, efficient hydrogen purification. Fuel Cell Store and Toyota Motor Corporation are exploring on-board and station-based hydrogen purification modules based on ceramic membrane technology, with field trials anticipated by late 2025. These systems can help address hydrogen purity requirements (ISO 14687), which are critical for fuel cell longevity and performance, especially in distributed and renewable hydrogen production scenarios.
Outlook: The next few years will likely see the first commercial installations of electroceramic membrane hydrogen separation systems, especially in industrial pilot plants and integrated energy projects. Challenges remain around long-term durability and scaling, but increased collaboration between materials suppliers, OEMs, and end-users is accelerating progress. As regulatory pressure mounts for low-carbon hydrogen and process intensification, the sector is expected to transition from pilot to early commercial phase by 2026-2027, with substantial opportunities across all major application segments.
Performance Metrics: Efficiency, Scalability, and Cost
Electroceramic membrane hydrogen separation systems are gaining increased attention in 2025 due to their potential for high efficiency, selectivity, and integration with renewable energy applications. Performance metrics such as hydrogen purity, permeation flux, system efficiency, scalability, and cost are central to evaluating their commercial viability and deployment potential in the next few years.
Efficiency remains a primary focus as research and pilot projects advance. Electroceramic membranes, such as those based on perovskite and doped barium cerate, can achieve hydrogen purities exceeding 99.9%, with selectivity against contaminants like CO2 and CH4 frequently surpassing 99% under optimized conditions. Recent tests by Hydrogenics and Siemens Energy demonstrate that integrated membrane modules can operate at temperatures between 600–900°C and reach hydrogen fluxes of 0.1–0.3 Nm3/m2h, depending on feed gas composition and pressure differentials.
System efficiency also hinges on energy consumption. Electroceramic membranes typically use less auxiliary energy compared to traditional pressure swing adsorption or cryogenic distillation, particularly when coupled with high-temperature processes such as steam methane reforming or biomass gasification. Demonstrator units from fuelcellmaterials and Haldor Topsoe report energy consumption figures below 2.5 kWh/kg H2 produced, positioning these systems as competitive for green and blue hydrogen production scenarios.
Scalability is progressing from lab to pilot and early commercial scales. Modular membrane arrays are being developed to handle from tens to several hundred Nm3/h hydrogen output. In 2024–2025, Elcogen has initiated field trials of stackable ceramic membrane modules designed for distributed hydrogen production at refueling stations and small industrial sites. Meanwhile, Honeywell is collaborating with chemical manufacturers to integrate larger, skid-mounted membrane systems for process gas upgrading, targeting capacities above 1,000 Nm3/h in the next few years.
Cost remains a challenge, though trends are positive. While current electroceramic membranes are more expensive than polymeric alternatives on a per-square-meter basis, ongoing improvements in materials engineering and manufacturing scale are expected to reduce costs by 20–30% through 2027, according to internal analyses by Haldor Topsoe. Early commercial installations project delivered hydrogen costs in the range of $2.5–$4/kg H2 depending on feedstock and scale, with further reductions anticipated as production volumes increase and system lifetimes are validated.
Overall, the next few years are crucial for validating the efficiency, scalability, and cost competitiveness of electroceramic membrane hydrogen separation systems, with multiple industry players actively advancing demonstration projects and commercial deployments worldwide.
Challenges and Barriers to Widespread Adoption
Electroceramic membrane hydrogen separation systems have garnered significant attention as promising technologies for efficient, selective, and high-purity hydrogen production. However, despite their technical potential, several challenges and barriers remain to their widespread adoption as of 2025 and in the coming years.
- Material Stability and Durability: A primary technical barrier is the long-term stability of electroceramic membranes under industrial operating conditions. Many promising materials, such as perovskite oxides, can degrade when exposed to contaminants like sulfur or carbon monoxide, or when subjected to thermal cycling. Companies such as Haldor Topsoe and Ceramatec are actively researching more robust compositions, but achieving consistent multiyear performance remains a challenge.
- Manufacturing Scale and Cost: The fabrication of dense, defect-free electroceramic membranes at scale is complex and costly. Processes such as tape casting and sintering require precise control, and raw materials like rare earth elements can be expensive. Manufacturers like CoorsTek and fuelcellmaterials are working to reduce costs and improve scalability, but current membrane modules are still significantly more expensive than incumbent pressure swing adsorption (PSA) or polymeric membrane technologies.
- Integration with Industrial Processes: Electroceramic hydrogen separation is most efficient at high temperatures, which presents integration challenges with existing infrastructure, particularly in refineries and ammonia plants. Retrofitting current systems, especially where lower-temperature operations are standard, requires significant capital investment and engineering adaptation (Air Liquide).
- System Complexity and Balance of Plant: These systems require sophisticated sealing, gas-tight interfaces, and heat management solutions to operate reliably. Any leaks or thermal inefficiencies can severely compromise hydrogen purity and system lifespan. Efforts by SINTEF and Haldor Topsoe highlight ongoing R&D into improved system designs and auxiliary components.
- Market Acceptance and Standardization: Widespread adoption is also hindered by the lack of industry standards specific to electroceramic membrane hydrogen separation. There is hesitancy among end-users to adopt unproven technologies over well-established alternatives, especially in safety-critical applications. Industry groups such as the U.S. Department of Energy Hydrogen and Fuel Cell Technologies Office are beginning to develop guidance and demonstration projects, but comprehensive standards are still in their infancy.
Looking ahead, continued progress in material science, cost reduction, and demonstration-scale deployment will be crucial. Overcoming these barriers will require coordinated efforts between technology developers, manufacturers, and end-users, alongside supportive policy frameworks to accelerate commercial adoption.
Regulatory Environment and Industry Standards
The regulatory environment for electroceramic membrane hydrogen separation systems is rapidly evolving as governments and international bodies intensify efforts to decarbonize energy systems and stimulate the adoption of clean hydrogen technologies. In 2025, the landscape is shaped by a mixture of updated hydrogen strategies, safety codes, and performance standards that directly influence the commercialization and deployment of these advanced separation membranes.
A key regulatory driver is the alignment of hydrogen purity requirements with end-use applications, such as fuel cell vehicles or industrial feedstocks. International standards, notably those developed by the International Organization for Standardization (ISO), are increasingly referenced in national regulations. Specifically, ISO 14687 sets the criteria for hydrogen quality, which electroceramic membrane developers must demonstrate their systems can consistently meet. In Europe, the CEN-CENELEC committees are actively harmonizing technical standards for hydrogen infrastructure, including separation technologies, under the European Clean Hydrogen Alliance framework.
Safety is another focal point. Organizations such as the U.S. Department of Energy (DOE) Hydrogen Program are updating safety guidelines to account for the unique operating conditions of high-temperature ceramic membranes. The International Electrotechnical Commission (IEC) is also expanding standards for the integration of hydrogen systems into industrial and grid applications, with input from industry stakeholders. Companies like Haldor Topsoe and CeramTec are active in regulatory consultations, advocating for protocols that recognize the specific safety profiles and operational limits of electroceramic materials.
Meanwhile, public funding programs increasingly require certification or independent validation against these standards. The European Union’s Clean Hydrogen Partnership has made compliance with ISO and CEN standards a precondition for project support in 2025 and beyond. Similar trends are emerging in Asia, with Japan’s Ministry of Economy, Trade and Industry (METI) updating technical guidelines to expedite the domestic deployment of hydrogen separation systems utilizing advanced ceramics.
Looking ahead, the industry anticipates further tightening of regulatory benchmarks, particularly concerning system durability, lifecycle environmental impacts, and interoperability with other hydrogen technologies. Industry groups and manufacturers are collaborating to establish new test protocols and accelerate standardization, aiming to position electroceramic membrane systems as a core enabler in the hydrogen value chain. This dynamic regulatory environment is expected to foster innovation while ensuring safety and reliability as these systems scale up toward commercial readiness.
Future Trends: Innovations, Investments, and Long-term Outlook
Electroceramic membrane hydrogen separation systems are emerging as a promising technology for efficient hydrogen purification and production, leveraging selective ion-conducting ceramics such as perovskite oxides and proton-conducting ceramics. As the hydrogen economy accelerates globally, significant innovations and investments are shaping the trajectory of this technology for 2025 and the coming years.
Recent developments focus on enhancing operational stability, scaling up module sizes, and reducing production costs. Leading manufacturers such as Haldor Topsoe and FuelCell Energy, Inc. are advancing solid oxide electrolysis and ceramic membrane modules designed for hydrogen separation at high temperatures. For instance, Haldor Topsoe’s SOEC (Solid Oxide Electrolyzer Cell) platforms are being expanded for industrial-scale hydrogen generation and purification, aiming for commercial deployments in 2025 and beyond.
On the innovation front, research initiatives are targeting new mixed ionic-electronic conducting (MIEC) materials to improve hydrogen flux and membrane durability. SINTEF has reported progress in developing robust ceramic membranes with enhanced selectivity and resistance to contaminants, critical for industrial applications such as ammonia production and refinery gas upgrading. Additionally, companies like Proton Energy Systems (NEL Hydrogen US) are collaborating with industrial partners to integrate electroceramic membrane systems into existing hydrogen supply chains, focusing on modularity and system integration for decentralized hydrogen hubs.
Investment trends indicate growing support from both government and private sectors to commercialize these technologies. The European Clean Hydrogen Alliance, which includes participants such as Air Liquide and Linde plc, is prioritizing membrane-based hydrogen separation as part of its strategic roadmap for green hydrogen infrastructure. Funding is increasingly directed toward pilot plants and demonstration projects, with several large-scale initiatives set to come online between 2025 and 2027.
Looking forward, the outlook for electroceramic membrane hydrogen separation systems is highly favorable. Market projections are buoyed by policies supporting low-carbon hydrogen and the anticipated demand in sectors requiring ultrapure hydrogen. The next few years are expected to see further reductions in membrane cost per unit area, improvements in system durability, and the first wave of commercial-scale deployments. As technical barriers are addressed and economies of scale are achieved, electroceramic membranes are positioned to play a central role in the evolution of the global hydrogen economy.
Sources & References
- Sumitomo Chemical
- NGK Insulators
- Shell
- Fraunhofer Society
- Elcogen
- CerPoTech
- Fraunhofer Institute for Ceramic Technologies and Systems
- Topsoe
- Air Liquide
- Siemens Energy
- Bosch
- Fuel Cell Store
- Toyota Motor Corporation
- fuelcellmaterials
- Elcogen
- Honeywell
- SINTEF
- U.S. Department of Energy Hydrogen and Fuel Cell Technologies Office
- International Organization for Standardization (ISO)
- CEN-CENELEC
- CeramTec
- Clean Hydrogen Partnership
- FuelCell Energy, Inc.
- Linde plc