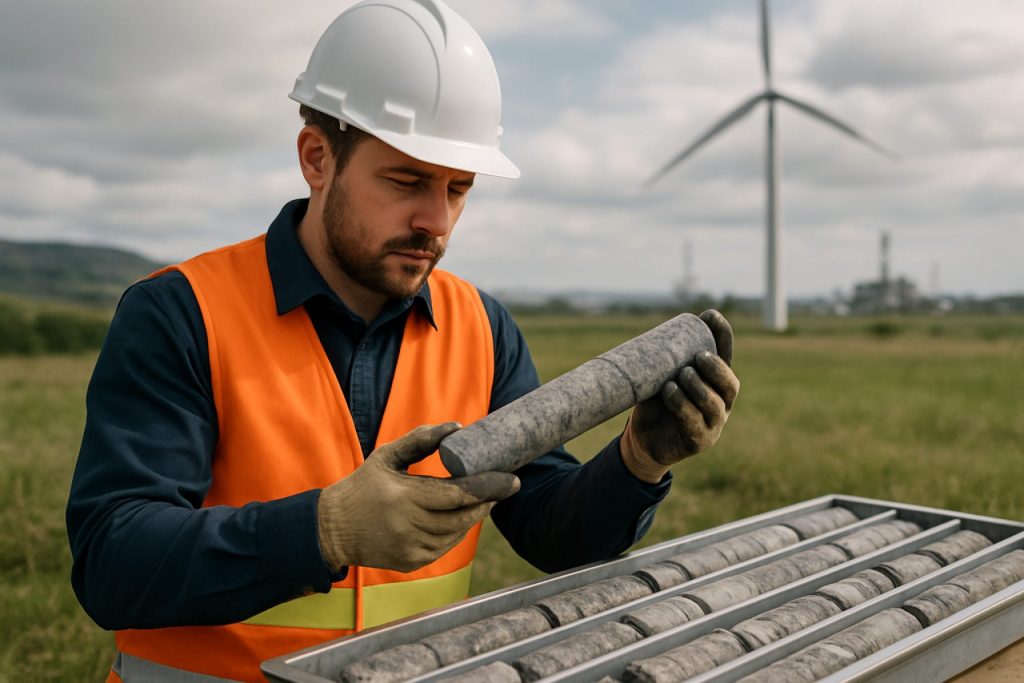
Table of Contents
- Executive Summary: Market Drivers and Key Insights for 2025
- The Science of Geological Core Sampling for Hydrogen Storage
- Current Global Landscape: Leading Projects and Companies
- Regulatory and Environmental Considerations in Core Sampling
- Technological Innovations Shaping Core Sampling Accuracy
- Market Size, Growth Projections & Investment Trends (2025–2028)
- Case Studies: Successful Hydrogen Storage Initiatives
- Challenges: Geological, Technical, and Economic Barriers
- Emerging Opportunities: New Applications and Business Models
- Future Outlook: The Next Wave of Subsurface Hydrogen Storage (2029 and Beyond)
- Sources & References
Executive Summary: Market Drivers and Key Insights for 2025
Geological core sampling is emerging as a cornerstone technology in the rapidly advancing market for subsurface hydrogen storage, directly supporting the global energy transition and decarbonization objectives for 2025 and beyond. The push for large-scale hydrogen storage is driven by the need to manage fluctuating renewable energy supplies and to ensure energy security, especially as nations work to meet ambitious net-zero targets. The ability to store hydrogen safely and efficiently underground—primarily in salt caverns, depleted hydrocarbon reservoirs, and aquifers—depends on a rigorous understanding of subsurface geology. This, in turn, demands high-quality core samples to inform site selection, risk assessment, and long-term monitoring strategies.
Key market drivers in 2025 include substantial public and private investments in hydrogen infrastructure, new regulatory frameworks, and the expansion of pilot and demonstration projects across Europe, North America, and Asia-Pacific. For instance, the European Union’s Hydrogen Strategy and the U.S. Department of Energy’s Hydrogen Shot initiative have both accelerated funding for hydrogen storage research, with core sampling at the heart of these efforts (U.S. Department of Energy). In 2024 and 2025, numerous geological surveys and demonstration projects—such as the UK’s HyNet North West and Germany’s H2CAST project—are actively utilizing advanced coring techniques to evaluate salt formations and depleted fields for hydrogen compatibility (HyNet North West; Technical University of Munich).
Technological innovation is a defining trend, with leading service companies developing specialized coring and core analysis methods to address unique hydrogen storage challenges. These include assessing caprock integrity, porosity, permeability, and geochemical interactions specific to hydrogen’s small molecular size and potential for embrittlement. Firms such as SLB (formerly Schlumberger) and Baker Hughes are deploying advanced wireline coring tools, real-time downhole sensors, and laboratory protocols tailored for hydrogen applications. Their work supports operators in derisking projects and achieving regulatory compliance.
Looking ahead, the outlook for geological core sampling in hydrogen storage remains robust. With over 20 commercial-scale hydrogen storage projects in the development pipeline for 2025–2028—many backed by major energy companies and government consortia—demand for specialized core sampling services is set to expand. Ongoing standardization efforts by organizations such as the IEA Greenhouse Gas R&D Programme (IEAGHG) and DNV are expected to further drive best practices, safety, and market confidence. In summary, core sampling is poised to play a pivotal role in unlocking the full potential of underground hydrogen storage as a critical enabler of the clean energy future.
The Science of Geological Core Sampling for Hydrogen Storage
Geological core sampling is an essential scientific process in the evaluation of subsurface formations for hydrogen storage, providing direct physical evidence of the rock types, porosity, permeability, and geochemical properties at depth. As the hydrogen economy advances through 2025 and beyond, core sampling technologies and methodologies are rapidly adapting to address the unique challenges posed by large-scale subsurface hydrogen storage, particularly in salt caverns, depleted oil and gas reservoirs, and deep saline aquifers.
The process typically begins with the drilling of exploratory boreholes at prospective storage sites. Cylindrical sections of rock, or “cores,” are retrieved and analyzed in laboratories for their mineralogy, pore structure, cap rock integrity, and reactivity with hydrogen. These analyses are pivotal in predicting how formations will behave during hydrogen injection, storage, and withdrawal cycles. For example, understanding the mineral composition of cap rocks is critical to assess potential hydrogen loss via diffusion or chemical reactions. In the last year, industry leaders such as SLB (formerly Schlumberger) and Baker Hughes have developed core analysis workflows specifically tailored for hydrogen storage, incorporating advanced imaging, tracer studies, and geochemical modeling.
Recent pilot projects across Europe and North America demonstrate the centrality of core sampling in project development. The Helmeth Hydrogen project in Germany, for instance, has highlighted the use of core samples to determine the suitability of salt formations for hydrogen storage and to inform cavern design and operational protocols. Similarly, Equinor‘s work on hydrogen storage in depleted gas reservoirs on the Norwegian Continental Shelf has involved extensive core retrieval and laboratory testing to validate the sealing capacity and long-term geochemical stability of the storage formations.
A key innovation for 2025 and the near future is the integration of digital core analysis with traditional laboratory methods. Companies like Core Laboratories are deploying high-resolution CT scanning, machine learning, and digital rock physics to predict hydrogen migration and storage capacity more efficiently, reducing the need for costly and time-consuming physical tests. These digital workflows are especially valuable for rapid screening of multiple storage sites and for optimizing storage strategies under variable operational scenarios.
Looking ahead, the outlook for geological core sampling in hydrogen storage is strong. As regulatory frameworks evolve and commercial-scale projects multiply, robust core sampling and analysis will remain indispensable for de-risking investments and ensuring long-term storage integrity. Collaboration between technology providers, operators, and research institutions is expected to yield further methodological advances, supporting the safe and effective deployment of subsurface hydrogen storage worldwide.
Current Global Landscape: Leading Projects and Companies
Geological core sampling has emerged as a foundational practice in the assessment and development of subsurface hydrogen storage, with growing momentum as countries and energy providers accelerate efforts to decarbonize energy systems in 2025 and beyond. Across Europe, North America, and parts of Asia-Pacific, pioneering projects and industry leaders are deploying advanced coring techniques to evaluate geologies suitable for safe, large-scale hydrogen containment.
In Europe, the Netherlands remains a key locus for innovation, leveraging its extensive depleted gas fields and salt caverns. Nederlandse Aardolie Maatschappij (NAM) and Shell are actively involved in geological characterization at sites like the Zuidwending salt caverns, employing continuous core recovery and advanced logging to verify rock integrity, porosity, and permeability for hydrogen injection and withdrawal cycles. The Gasunie HyStock project, officially endorsed by the Dutch government, has been conducting core sampling in support of its planned large-scale hydrogen storage facility, aiming to deliver operational capacity by the late 2020s.
The United Kingdom is advancing geological core sampling through its British Geological Survey in collaboration with energy majors such as Equinor and Centrica. Current (2025) core sampling campaigns in the East Yorkshire region and the North Sea are focused on understanding cap rock integrity and storage potential in both aquifers and legacy hydrocarbon fields. Equinor’s Hydrogen to Humber project, for example, integrates extensive coring of Permian-age salt strata to validate its suitability for high-pressure hydrogen storage.
In North America, the United States Department of Energy’s Hydrogen Shot initiative is driving core sampling in Gulf Coast salt domes and depleted fields, with public-private partnerships involving Chevron and ExxonMobil currently underway. These efforts emphasize tailored coring protocols to assess potential hydrogen embrittlement and geochemical interactions under storage conditions.
Australia is also progressing with core sampling projects led by CSIRO and the Australian Gas Infrastructure Group (AGIG) in South Australia’s salt basins, preparing the groundwork for future commercial hydrogen storage hubs.
Looking ahead, the next few years are expected to see a sharp increase in core sampling activities as regulatory frameworks tighten and project developers seek to derisk investments. Companies are investing in automated coring technologies and digital core analysis, positioning geological core sampling as a crucial enabler for the global scale-up of subsurface hydrogen storage.
Regulatory and Environmental Considerations in Core Sampling
Geological core sampling for subsurface hydrogen storage in 2025 is increasingly shaped by stringent regulatory frameworks and heightened environmental scrutiny. Regulatory agencies worldwide are updating standards to reflect the unique challenges posed by hydrogen storage, such as potential subsurface migration, integrity of caprocks, and risks of induced seismicity. Core sampling is critical for characterizing formation lithology, porosity, permeability, and geochemical compatibility, all necessary for regulatory approval of storage sites.
In the European Union, the European Geosciences Union highlights that the EU’s revised Gas Directive (expected to take effect by 2025) will include specific provisions for hydrogen storage, emphasizing robust site characterization via core analysis. Operators must demonstrate that sampled cores confirm the presence of impermeable caprocks and absence of geohazards before permits are issued. Similar requirements are detailed in the UK’s regulatory guidance, with the Environment Agency mandating comprehensive core-based site assessments and long-term monitoring plans as part of permit applications.
In the United States, the U.S. Department of Energy (DOE) Office of Clean Energy Demonstrations is funding pilot projects in salt caverns and depleted reservoirs, demanding core sampling campaigns that meet standards set by the American Petroleum Institute (API). These standards include guidelines for preserving core integrity, sampling frequency, and laboratory testing to assess caprock reaction to hydrogen exposure. Environmental impact assessments must address potential groundwater contamination and methane mobilization, with core data providing foundational evidence.
Environmental considerations are also driving the adoption of less invasive core sampling techniques and improved waste handling protocols. Companies such as SLB (Schlumberger) and Baker Hughes report increased deployment of low-footprint coring tools and environmentally benign drilling fluids to minimize site disturbance. These measures align with evolving standards from organizations like the International Organization for Standardization (ISO), which is developing new technical specifications for hydrogen storage site assessment.
Looking ahead, regulatory harmonization across jurisdictions is anticipated as hydrogen storage scales up. Industry stakeholders are calling for unified core sampling and reporting protocols to streamline cross-border projects and accelerate permitting, a trend that groups such as the Hydrogen Europe are actively promoting. The outlook for 2025 and beyond is one of increased oversight, technological innovation, and collaborative standard-setting to ensure safe, environmentally responsible subsurface hydrogen storage.
Technological Innovations Shaping Core Sampling Accuracy
Technological advancements are rapidly enhancing the accuracy and efficiency of geological core sampling for subsurface hydrogen storage, a sector experiencing significant momentum as hydrogen emerges as a key component in global decarbonization strategies. Recent innovations are being driven by the need to precisely characterize potential storage sites, evaluate caprock integrity, and predict long-term hydrogen behavior in subsurface formations.
In 2025, digitalization and automation are transforming traditional coring methods. Companies such as SLB (Schlumberger) have introduced new coring tools integrated with advanced sensors, enabling real-time data acquisition during the drilling process. These tools collect not only high-quality core samples but also continuous downhole measurements—such as pressure, temperature, and formation gas content—crucial for assessing hydrogen migration and storage capacity.
Moreover, the use of Baker Hughes‘ wireline formation testing and core analysis technologies is enhancing the understanding of rock-fluid interactions specific to hydrogen. Their systems allow for pressurized coring, which preserves in-situ conditions and minimizes sample alteration, yielding more representative data for permeability and porosity analyses. These parameters are essential for modeling hydrogen injectivity, containment, and retrieval.
High-resolution, non-invasive imaging techniques are also seeing increased adoption. Weatherford International is applying computed tomography (CT) and nuclear magnetic resonance (NMR) imaging to extracted cores. These imaging modalities provide detailed three-dimensional views of pore networks and mineral structures without physically altering the samples, allowing for more accurate assessments of storage potential and caprock effectiveness.
Additionally, the integration of artificial intelligence and machine learning is optimizing core sample interpretation. Companies like Halliburton are deploying cloud-based platforms that aggregate core data with seismic, petrophysical, and geological models. This data fusion enables more robust predictions of subsurface hydrogen behavior, facilitating improved site selection and risk mitigation strategies.
Looking ahead, the sector anticipates further convergence of robotics, remote operation, and real-time analytics. The deployment of autonomous coring systems is expected to reduce human exposure to hazardous environments while increasing sampling precision. As regulatory frameworks for hydrogen storage solidify in the coming years, such innovations will be indispensable for verifying site suitability and supporting large-scale hydrogen infrastructure deployment.
Market Size, Growth Projections & Investment Trends (2025–2028)
The global market for geological core sampling tailored to subsurface hydrogen storage is poised for significant growth during 2025–2028, propelled by the acceleration of hydrogen as a cornerstone in global decarbonization strategies. Core sampling enables detailed assessment of geomechanical, mineralogical, and petrophysical properties of candidate storage formations, which is fundamental for safe, efficient underground hydrogen storage. As countries and energy majors commit to scaling up hydrogen infrastructure, demand for advanced core analysis services and technologies is rising.
The emerging market is heavily concentrated in Europe, North America, and parts of Asia-Pacific, where national hydrogen strategies and pilot projects drive activity. The European Union’s REPowerEU plan, targeting 50 million tonnes of renewable hydrogen consumption by 2030, is stimulating investment in hydrogen storage pilot projects and associated geological studies Shell. For example, the Hystories and HyUsPRe projects are leveraging core sampling to evaluate depleted gas reservoirs and salt caverns for hydrogen storage potential, with Shell and other partners conducting extensive core retrieval and lab analysis in the North Sea and continental Europe TotalEnergies.
In the United States, the Department of Energy’s Hydrogen Shot Initiative is funding demonstration-scale storage projects, with a strong emphasis on subsurface characterization through core sampling and testing U.S. Department of Energy. Key service providers such as SLB (Schlumberger) and Baker Hughes are reporting increased contract awards for coring, core analysis, and digital core simulation services, especially in regions with depleted oil and gas fields suitable for conversion.
From 2025 to 2028, market projections indicate a compound annual growth rate (CAGR) exceeding 12% for geological services linked to hydrogen storage, outpacing traditional hydrocarbon-related coring due to the higher technical requirements and regulatory scrutiny for hydrogen integrity and containment. Investment is flowing into both hardware—advanced coring tools, real-time downhole sensors—and software for digital core analysis, with Halliburton and Core Laboratories introducing new solutions tailored for hydrogen compatibility and rock-hydrogen interaction studies.
- Europe: Large-scale pilot projects and government-backed hydrogen valleys are driving multi-million-euro investments in core sampling and geocharacterization.
- USA: New federal awards and private-sector partnerships are expanding commercial core sampling campaigns in key basins.
- Asia-Pacific: Australia and Japan are initiating feasibility studies, with local firms collaborating with international core analysis specialists.
Looking ahead, the market is expected to mature rapidly, with core sampling becoming a standard prerequisite for all major underground hydrogen storage development. This trajectory is reinforced by increasing regulatory requirements and the need for robust, bankable subsurface data to secure project financing and insurance.
Case Studies: Successful Hydrogen Storage Initiatives
Geological core sampling is emerging as a foundational step in the assessment and deployment of subsurface hydrogen storage initiatives. In 2025 and the upcoming years, several high-profile projects across Europe and North America are showcasing the pivotal role that core sampling plays in derisking geological formations and optimizing storage strategies.
One notable example is the pilot project by RWE Gas Storage West in Germany, which began in 2024 and continues development in 2025. Here, extensive core sampling of salt caverns in the Epe site near Gronau is being used to characterize the petrophysical and geomechanical properties of the salt rock. These efforts are critical for assessing the suitability of the caverns for hydrogen storage, as well as monitoring any potential interactions between hydrogen and the host rock. RWE’s pilot is among the first in Germany to transition a natural gas cavern to pure hydrogen, with core analysis underpinning their safety and sealing evaluations.
Similarly, the United Kingdom’s Rough Storage site, operated by Centrica, is leveraging historic core samples and new drilling campaigns to assess reservoir compatibility with hydrogen injection and withdrawal. The Rough site, previously a natural gas storage facility in the North Sea, is the focus of a planned conversion to store up to 1.5 TWh of hydrogen by 2028. The project’s core sampling program is focused on evaluating caprock integrity and the chemical reactivity of reservoir rocks when exposed to hydrogen, aiming to prevent leakage and maintain long-term storage security.
In the United States, the SoCalGas 'Angeles Link' project in California is conducting core sampling of depleted gas reservoirs and saline aquifers to build a regional hydrogen hub. Their 2025 work program emphasizes advanced coring technologies to retrieve undisturbed samples, enabling laboratory testing of hydrogen diffusion, mineral alteration, and microbial activity in the subsurface. These data are shaping regulatory submissions and investment decisions for future commercial-scale storage.
Looking ahead, industry bodies such as the European Energy Research Alliance (EERA) are coordinating multinational research on core sampling best practices, aiming to standardize protocols across different lithologies and storage types. Results from these case studies are expected to inform emerging projects in the Netherlands, Denmark, and Canada, where geological core sampling will remain a prerequisite for large-scale, safe, and sustainable hydrogen storage.
Challenges: Geological, Technical, and Economic Barriers
Geological core sampling is at the heart of assessing and de-risking sites for subsurface hydrogen storage, but the process faces a unique set of challenges in the context of hydrogen’s distinct properties and the nascent status of large-scale hydrogen storage projects. As the hydrogen economy accelerates into 2025 and beyond, overcoming geological, technical, and economic barriers in core sampling is crucial for project feasibility and safety assurance.
One of the primary geological challenges is the accurate characterization of caprock integrity and reservoir quality under hydrogen cycling conditions. Unlike natural gas, hydrogen molecules are significantly smaller and more diffusive, raising concerns about potential leakage through microfractures or previously undetected faults. Core sampling must therefore achieve exceptionally high recovery rates and minimal disturbance to accurately gauge rock porosity, permeability, and caprock sealing capacity. Companies such as SLB (Schlumberger) and Baker Hughes have reported that adapting core retrieval and preservation techniques to minimize oxidation or hydrogen loss during transport and analysis remains a major technical hurdle.
Laboratory testing protocols for hydrogen-rock interactions also present technical challenges. Hydrogen can react with certain minerals or induce microbial activity that may compromise reservoir performance over time. As noted by Shell, experimental setups must now simulate cyclic hydrogen injection and withdrawal, including high-pressure and variable temperature conditions, to better predict reservoir behavior. This increases both the technical complexity and the cost of core analysis, as specialized equipment and containment systems are required to handle hydrogen safely and prevent contamination.
Economically, the costs associated with core drilling and advanced laboratory analysis are significant. The need for high-frequency sampling and tailored core handling protocols for hydrogen, as opposed to conventional hydrocarbons, can raise project appraisal budgets by 20–40%. Only a handful of service providers currently offer hydrogen-specific core analysis protocols, limiting competition and driving up costs. According to Equinor, economic uncertainties around the long-term viability of hydrogen storage—especially in newly explored geological formations—make it difficult to justify the upfront investment without clear regulatory incentives or long-term offtake agreements.
Looking ahead, ongoing demonstrations and pilot projects are expected to yield critical data to refine core sampling methodologies. Industry leaders are collaborating with academic institutions to develop standardized testing procedures and accelerate regulatory acceptance. However, until core sampling technologies and workflows are fully tailored to hydrogen’s unique characteristics, geological, technical, and economic barriers will continue to shape the pace and scale of subsurface hydrogen storage deployment through the remainder of this decade.
Emerging Opportunities: New Applications and Business Models
As the global transition to low-carbon energy systems accelerates, geological core sampling is emerging as a critical enabler for the safe and effective development of subsurface hydrogen storage projects. In 2025 and the coming years, the sector is witnessing a surge in new applications and business models driven by the need to repurpose geological formations for large-scale, seasonal hydrogen storage—a key requirement for grid balancing and decarbonizing heavy industry.
Core sampling techniques, traditionally applied in hydrocarbon exploration, are swiftly being adapted for hydrogen-specific challenges. Operators are now prioritizing detailed mineralogical, petrophysical, and microbiological analyses to understand how hydrogen interacts with reservoir rocks, caprocks, and potential contaminants. Such insights are crucial to assess risks like hydrogen embrittlement, microbial consumption, and caprock integrity, all of which directly influence project bankability and regulatory approval.
Several energy majors and service companies are actively deploying advanced coring and testing technologies. For example, SLB (formerly Schlumberger) has announced the expansion of its core analysis services to include hydrogen compatibility testing, leveraging its global network of laboratories to support hydrogen storage projects in Europe and North America. Concurrently, Baker Hughes is investing in new core acquisition and preservation methods to maintain hydrogen purity and minimize sample alteration during extraction and transport.
Emerging business models are focusing on integrated service offerings: from site screening and core sampling to reservoir simulation and regulatory compliance. Companies such as Storegga are collaborating with technology providers and storage operators to deliver turnkey solutions, enabling faster de-risking and commercialization of storage assets. Meanwhile, public-private consortiums, including members of the Hydrogen Energy Supply Chain, are funding pilot projects that generate open-access core data to accelerate industry-wide learning and standardization.
Looking ahead, digitization and automation are poised to further transform core sampling. Real-time downhole data acquisition, AI-driven sample analysis, and digital twin modeling are expected to streamline project timelines and reduce costs. The expansion of hydrogen storage hubs in strategic locations—such as North Sea salt caverns and depleted gas fields in the U.S. Gulf Coast—will continue to stimulate demand for specialized core sampling expertise and foster new partnerships between storage developers, technology firms, and local utilities.
Overall, the next few years will see geological core sampling evolve from a technical necessity to a strategic business lever, underpinning the rapid scaling of subsurface hydrogen storage as part of the global energy transition.
Future Outlook: The Next Wave of Subsurface Hydrogen Storage (2029 and Beyond)
As the hydrogen economy advances, geological core sampling is poised to play an increasingly critical role in shaping the future of subsurface hydrogen storage, especially beyond 2029. By 2025, several pioneering projects and technology developments are setting a foundation for the next wave of exploration, risk assessment, and operational optimization in this emerging sector.
Core sampling provides direct physical evidence from targeted geological formations, enabling accurate evaluation of porosity, permeability, mineralogy, and sealing capacities—parameters essential for determining a site’s suitability for hydrogen storage. Recent years have seen expanded collaboration between energy companies, drilling technology providers, and research institutions to refine core acquisition and analysis techniques specifically for hydrogen as opposed to traditional hydrocarbons or CO2.
In 2025, organizations like SLB (Schlumberger) and Baker Hughes are expected to roll out enhanced coring methods and digital core analysis workflows tailored for the unique challenges of hydrogen storage. These advancements include improved downhole coring tools capable of minimizing core disturbance and advanced laboratory protocols to characterize potential hydrogen-rock interactions, such as mineral alteration and microbial activity, over long timescales.
Large-scale demonstration projects in Europe, such as those coordinated by RWE and Equinor, are integrating comprehensive core sampling campaigns into their site qualification processes for planned hydrogen storage in depleted gas fields and salt caverns. These programs are generating extensive datasets that not only inform immediate project viability but also feed into the development of industry-wide best practices and regulatory standards for hydrogen containment security and environmental monitoring.
Looking toward 2029 and beyond, the accumulation and digitization of core-derived data are expected to enable more predictive modeling of hydrogen migration, storage integrity, and the identification of globally scalable storage sites. The trend toward automation and real-time analytics in core processing—supported by investments from companies such as Halliburton—will further accelerate the de-risking and deployment of commercial-scale subsurface hydrogen storage.
As governments and industry bodies, including the International Energy Agency (IEA), emphasize the rapid build-out of hydrogen infrastructure, the importance of robust geological core sampling will only increase. By the end of the decade, best-in-class core sampling practices are likely to be codified into international standards, underpinning investor confidence and public acceptance of hydrogen as a safe and sustainable energy vector.
Sources & References
- HyNet North West
- SLB
- Baker Hughes
- IEA Greenhouse Gas R&D Programme (IEAGHG)
- DNV
- Equinor
- Core Laboratories
- Nederlandse Aardolie Maatschappij (NAM)
- Shell
- Gasunie
- ExxonMobil
- CSIRO
- Australian Gas Infrastructure Group (AGIG)
- European Geosciences Union
- Environment Agency
- American Petroleum Institute (API)
- International Organization for Standardization (ISO)
- Hydrogen Europe
- Weatherford International
- Halliburton
- TotalEnergies
- European Energy Research Alliance (EERA)
- SLB (Schlumberger)
- Storegga
- International Energy Agency (IEA)